Genome Editing Demystified: Past, Present, And Future
sabeeh
on
August 12, 2024
Overview
Gene editing (also called as genome editing) is a scientific method that allows for precisely altering an organism’s DNA. This innovative technology enables scientists to modify, delete, or insert specific genes or smaller DNA elements within an organism’s genome, thus influencing its characteristics or behavior. The ability to directly manipulate genes holds significant potential for various applications, including medical treatments, agriculture, and biological research.
Gene editing is particularly valuable in studying diseases, developing new therapies, and improving crop resilience. Unlike traditional genetic engineering techniques, which often involve transferring genes from one organism to another, gene editing directly modifies the organism’s DNA.
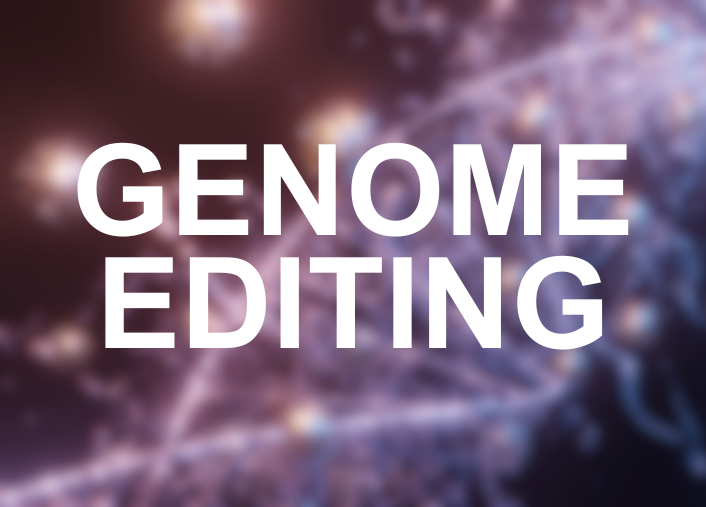
CRISPR Gene Editing
CRISPR gene editing, a specific form of gene editing, revolutionized the field with its precision, simplicity, and efficiency. CRISPR, which stands for Clustered Regularly Interspaced Short Palindromic Repeats, is a natural system that bacteria use to defend against viruses. Scientists have harnessed this system to create a powerful tool for editing genes.
The CRISPR system includes two key components: the Cas9 protein, which acts like molecular scissors to cut DNA, and a piece of RNA that guides Cas9 to the specific DNA sequence to be edited. This technology allows for targeted alterations to an organism’s genetic code, enabling researchers to study gene function and develop treatments for genetic disorders.
Gene editing generally involves identifying the target DNA sequence and then using a gene-editing tool like CRISPR to cut the DNA precisely at that location. Once the DNA is cut, the cell’s natural repair mechanisms are triggered. Scientists can exploit these repair processes to disable a gene, correct a genetic mutation, or insert a new gene.
In the case of CRISPR, the guide RNA is designed to match the target DNA sequence. When the Cas9 protein, guided by this RNA, reaches the target site, it creates a precise cut in the DNA. The cell then repairs this cut by simply joining the ends back together (which can disrupt the gene’s function) or by using a supplied piece of DNA as a template for repair, introducing new genetic material.
Ethics
The ethical implications of gene editing are a topic of significant debate. While the potential benefits are vast, including treating hereditary diseases, improving food security, and advancing scientific understanding, there are also concerns. Ethical considerations include the potential for unintended consequences, such as off-target effects where the wrong part of the genome is edited, and the long-term impacts on ecosystems and biodiversity.
Additionally, there are concerns about gene editing in humans, particularly regarding germline editing, which would affect future generations. The possibility of creating “designer babies” with selected traits raises social, moral, and ethical questions. As such, the ethical use of gene editing requires careful consideration, regulation, and public dialogue to balance its benefits against the risks and ethical dilemmas it presents.
A Brief History
The first successful gene editing effort, directly altering the DNA within a living organism, can be traced back to the early 1990s. A key milestone was achieved in 1994 when researchers at the National Institutes of Health in the United States successfully performed gene therapy on a four-year-old girl. This case involved the use of gene editing to treat a rare genetic disorder known as severe combined immunodeficiency (SCID), also known as “bubble boy disease.”
In this groundbreaking effort, scientists used a retrovirus as a vector to deliver a corrected gene into the girl’s white blood cells. The introduced gene was intended to correct the genetic defect causing her SCID. This treatment was not a permanent cure, as it only edited the white blood cells, not the stem cells that continually produced new blood cells. However, it marked a significant early success in gene editing and gene therapy, demonstrating the potential of these types of techniques to treat genetic disorders.
This event was a precursor to more sophisticated gene editing techniques that emerged later, such as those utilizing Zinc Finger Nucleases (ZFNs), TALENs (Transcription Activator-Like Effector Nucleases), and eventually CRISPR-Cas9. These subsequent developments offered more precision, efficiency, and versatility in gene editing, greatly expanding the technology’s potential applications in research and medicine.
Developments
The very first successful gene editing experiments date back to the 1970s and 1980s, with the development of recombinant DNA technology. This groundbreaking work laid the foundation for modern gene editing. However, “successful” can be interpreted in various ways depending on the specific context or the milestone.
Here are a few key developments:
Recombinant DNA Technology (1970s-1980s): Scientists Herbert Boyer and Stanley Cohen achieved a breakthrough in 1973 by creating the first recombinant DNA organisms. This was done by cutting and combining DNA from different organisms, a precursor to more targeted gene editing techniques.
Gene Targeting in Mice (1989): A significant early success in gene editing was the demonstration of gene targeting in mice by Mario Capecchi, Martin Evans, and Oliver Smithies. They developed techniques for altering the DNA of mice, a process that earned them the Nobel Prize in Physiology or Medicine in 2007. This work involved modifying specific genes in mouse embryonic stem cells, which were then used to create genetically modified mice.
Zinc Finger Nucleases (Early 2000s): Developing zinc finger nucleases (ZFNs) allowed for more precise gene editing. ZFNs are engineered proteins that can cut DNA at specific locations, enabling targeted genetic modifications. This technology was a step forward in the precision and efficiency of gene editing.
CRISPR-Cas9 (2012): A major milestone was the adaptation of the CRISPR-Cas9 system for gene editing in 2012 by Jennifer Doudna and Emmanuelle Charpentier, among others. This technology allowed for easier, more precise, and more efficient editing of genes in a wide range of organisms, including humans. The first successful applications of CRISPR-Cas9 in human cells were demonstrated shortly after its discovery.
Clinical Applications (2010s): In the late 2010s, gene editing began to be used in clinical trials. One notable example is using CRISPR-Cas9 for treating genetic disorders like sickle cell anemia and β-thalassemia. These trials marked the first instances of CRISPR successfully treating human genetic diseases.
Each milestone represents a significant advance in gene editing, contributing to our current understanding and capabilities in genetic engineering.
Recent Breakthroughs In CRISPR Technology
Recent advances in CRISPR-Cas9 technology have led to significant breakthroughs in various fields, particularly medicine and biology. A few key developments include:
Applications in Cardiovascular Research
CRISPR-Cas9’s expansion, including emerging CRISPR-associated protein (Cas) nucleases and novel applications, has substantially impacted cardiovascular research. This includes generating genetically modified models for cardiovascular diseases and applying CRISPR technologies to treat different cardiovascular conditions.
Cell Therapy
The CRISPR toolbox has evolved into three generations of genome editing tools: nucleases, base editors, and prime editors. These tools are applied in cell therapy, where cells are isolated, edited ex vivo using CRISPR, and reintroduced into the patient. This approach has implications for treating various diseases, including cancer.
Therapeutic Genome Editing
CRISPR-Cas9 has shown promise in therapeutic strategies, with potential applications in various types of gene therapy.
Biological and Biomedical Applications
CRISPR-Cas9 and Cas13a systems are being adapted for various applications, including diagnostics, gene therapy, live-cell imaging, genome screening, and cancer research. These technologies provide new ways to address biological questions and medical challenges, such as antibiotic-resistant bacteria and RNA virus replication.
Cancer Immunotherapy
CRISPR-Cas9 is shaping into an effective tool in enhancing cancer immunotherapy. It is being used to identify new targets for cancer treatment, potentially improving the efficacy of immunotherapies by editing genes involved in cancer progression and immune evasion.
These breakthroughs illustrate the versatility and potential of CRISPR-Cas9/Cas13a in advancing medical science and treating diseases. The technology is evolving rapidly, promising to bring more innovative solutions to healthcare and research in the near future.
The Future Of Gene Editing
The future of gene editing holds immense potential across various fields, from medicine to agriculture and beyond. As the technology continues to advance, we can anticipate several key developments and trends:
Medical Treatments and Cures
One of the most promising applications of gene editing lies in its potential to treat and cure genetic diseases. Diseases like cystic fibrosis, sickle cell anemia, and certain forms of cancer, which have a clear genetic basis, are prime candidates for gene editing therapies. As our understanding of the genetic basis of diseases improves, gene editing could be used to correct these genetic defects directly.
Precision and Safety Improvements
Ongoing research will likely make gene editing tools like CRISPR more precise and safe. Reducing off-target effects – where the wrong part of the genome is edited – is a key area of focus. Improved precision will enhance the safety and efficacy of gene editing applications, particularly in clinical settings.
Regenerative Medicine
Gene editing could play a significant role in regenerative medicine, including tissue engineering and organ regeneration. By precisely manipulating genes, growing organs for transplantation or repairing damaged tissues might be possible, significantly impacting healthcare.
Agricultural Advancements
In agriculture, gene editing offers the potential to create crops that are more nutritious, resistant to pests and diseases, and able to withstand environmental stressors like drought or extreme temperatures. This could lead to increased food security and sustainability in farming.
Ethical and Regulatory Developments
As gene editing technology progresses, ethical considerations and regulatory frameworks will evolve. This includes policies around human germline editing, which has implications for future generations, and guidelines for gene editing in animals and plants.
Gene Drives and Environmental Impact
Gene editing could be used to develop gene drives, which promote the rapid spread of genetic modifications through a population. This could have significant applications in controlling vector-borne diseases like malaria but also raises ecological and ethical questions.
Personalized Medicine
The future of gene editing in personalized medicine, where treatments are tailored to the individual’s genetic makeup, is promising. This could lead to more effective and less invasive treatments for various conditions.
References
- https://pubmed.ncbi.nlm.nih.gov/36895064/
- https://pubmed.ncbi.nlm.nih.gov/37193354/
- https://pubmed.ncbi.nlm.nih.gov/37027099/
- https://pubmed.ncbi.nlm.nih.gov/35705772/
- https://pubmed.ncbi.nlm.nih.gov/36797756/
- https://www.ncbi.nlm.nih.gov/pmc/articles/PMC2907101/
- https://www.genome.gov/about-genomics/policy-issues/Genome-Editing/ethical-concerns
- https://medlineplus.gov/genetics/understanding/genomicresearch/genomeediting/
- https://americanhistory.si.edu/collections/object-groups/birth-of-biotech/recombinant-dna-in-the-lab
- https://innovativegenomics.org/education/digital-resources/what-is-crispr/
- https://www.nejm.org/doi/full/10.1056/NEJMoa2031054
*Understanding your genetics can offer valuable insights into your well-being, but it is not deterministic. Your traits can be influenced by the complex interplay involving nature, lifestyle, family history, and others.
Our reports have not been evaluated by the Food and Drug Administration. The contents on our website and our reports are for informational purposes only, and are not intended to diagnose any medical condition, replace the advice of a healthcare professional, or provide any medical advice, diagnosis, or treatment. Consult with a healthcare professional before making any major lifestyle changes or if you have any other concerns about your results. The testimonials featured may have used more than one LifeDNA or LifeDNA vendors’ product or reports.